Urban Forests Unveiled: How London’s Trees Are Battling Carbon Emissions


As the morning fog lifts, unveiling the ever-evolving skyline of London, a silent yet formidable force is at work as amidst the hum of the city, London’s trees are locked in a relentless battle. The battle is not waged with swords or speeches but with leaves and branches. London’s trees — steadfast sentinels rooted in the city’s parks, streets, and squares — stand on the frontline against the planet’s invisible foe: carbon emissions.
In a year, a single mature tree can absorb as much as 48 pounds of carbon dioxide, making the city’s urban forest a crucial frontline against climate change. Yet, not all boroughs are equally armed in this fight.1 Our analysis reveals stark differences: Consider this: In just one year, the trees of Enfield, one of London’s leafiest boroughs, sequestered over an estimated 80 million kilograms of carbon dioxide. That’s equivalent to the annual emissions from approximately 17,400 cars. This isn’t just a number; it’s a testament to the power of urban forests and their pivotal role in shaping the health of a city.
These leafy giants are more than just city embellishments; they are the unsung heroes in London’s strategy to cut down its annual carbon footprint, which stands at a staggering 19 million tonnes.
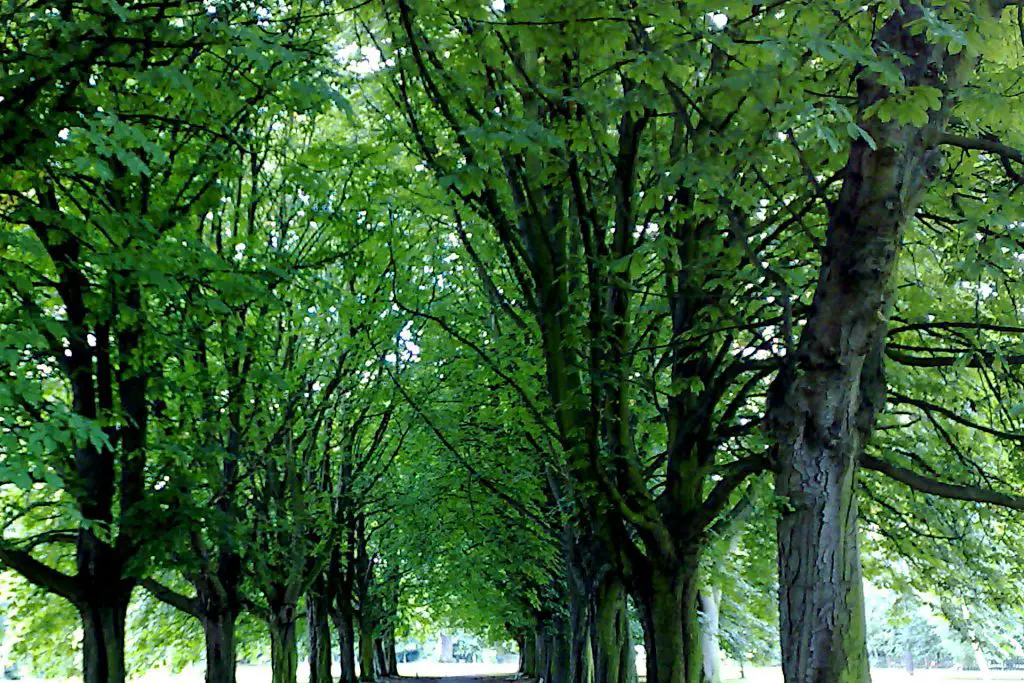
London’s urban forests stand as unsung heroes in the fight against climate change. These verdant spaces are more than mere pockets of tranquility; they are vital ecosystems that provide a natural bulwark, absorbing carbon emissions through a process known as carbon sequestration. The city’s diverse array of trees serves as a living carbon sink, quietly converting the carbon dioxide that cloaks our cities into life-giving oxygen and solid carbon stored within their trunks, roots, and leaves.
Amid the myriad of technological advancements heralded as beacons of hope for sustainable futures, trees remain one of the most efficient, cost-effective solutions to urban pollution. They are the lungs of the city, often overlooked yet indispensable in enhancing air quality, cooling sweltering cityscapes, and fostering biodiversity.
This study unfolds the narrative of London’s leafy guardians, examining how species from the mighty oak to the humble hawthorn are instrumental in mitigating greenhouse gases. While London’s trees stand as a collective force, the sequestration power varies widely across the city’s tapestry of boroughs. By delving into recent datasets,2 excluding regions with incomplete data such as Brent, Croydon, Havering, Hackney, and Wandsworth, a comprehensive analysis emerges, showcasing the unsung ecological services these urban canopies provide.
The Carbon Sequestration Machinery: Understanding the Basics
The carbon sequestration machinery operates on a simple, yet profound principle: trees absorb carbon dioxide (CO2) through the process of photosynthesis, converting it into organic carbon and releasing oxygen back into the atmosphere. In the grand tapestry of the urban ecosystem, each tree acts as a mini reservoir, partaking in this carbon exchange. The leaves act as panels absorbing sunlight, driving the chemical reactions that transform CO2 into glucose, the basic energy currency of a tree.
The role of urban trees in the carbon cycle can be encapsulated in three points:
- Carbon Uptake: Trees in urban settings draw in CO2 from their immediate surroundings, where traffic and buildings are significant sources of emissions.
- Carbon Storage: The biomass of trees — their trunks, branches, leaves, and roots — stores carbon for decades, sometimes centuries, sequestering it away from the atmosphere.
- Oxygen Release: By releasing oxygen back into the urban atmosphere, trees contribute to a healthier air quality, mitigating the impact of pollutants.
In urban settings, where greenhouse gas emissions are prolific, the role of carbon sequestration is both vital and multi-faceted. Urban forests—comprising street trees, park trees, and private gardens—form a green canopy that serves as the city’s lungs. These areas are not just passive landscapes but active climate regulators. They absorb CO2, one of the primary greenhouse gases, from car exhausts, heating systems, and industrial activities, helping to stabilize urban microclimates and offsetting the urban heat island effect.
To conceptualize the disparity in carbon sequestration across London, consider a comparative table of tree density and species composition across selected boroughs:
Borough | Tree Density (per sq mile) | Species Composition |
---|---|---|
Islington | 6,322 | High diversity |
Bexley | 500 | Moderate diversity |
Camden | 2,878 | Selected high-absorbing species |
Waltham Forest | 2,861 | Diverse with many young trees |
These figures are not just numbers; they represent the frontline of urban defense against climate change. As London grapples with its commitment to the Paris Agreement and aims for net-zero emissions, the carbon budget of its urban forests could be a game-changer.
To illustrate the magnitude of their impact, consider the following:
- A single mature tree can absorb approximately 22 kilograms of CO2 annually — the equivalent of driving a car for 25,000 miles.
- In one year, London’s urban trees are estimated to remove 2.4 million tons of air pollution, including CO2.
The benefits of urban trees extend beyond carbon sequestration, encompassing water management through interception of rainfall, soil conservation, and providing social benefits like improved mental health for city residents.
In truth, the urban canopy is a complex but efficient machinery, one that London must optimize to meet its ambitious environmental targets. Understanding the basics of carbon sequestration is not just academic—it’s a crucial step in planning for a sustainable, resilient cityscape. As trees emerge as silent yet formidable players in carbon mitigation, the data not only underscores the importance of this natural process but also provides a blueprint for enhancing the city’s carbon management with green infrastructure.
Factors Influencing Carbon Sequestration in Trees
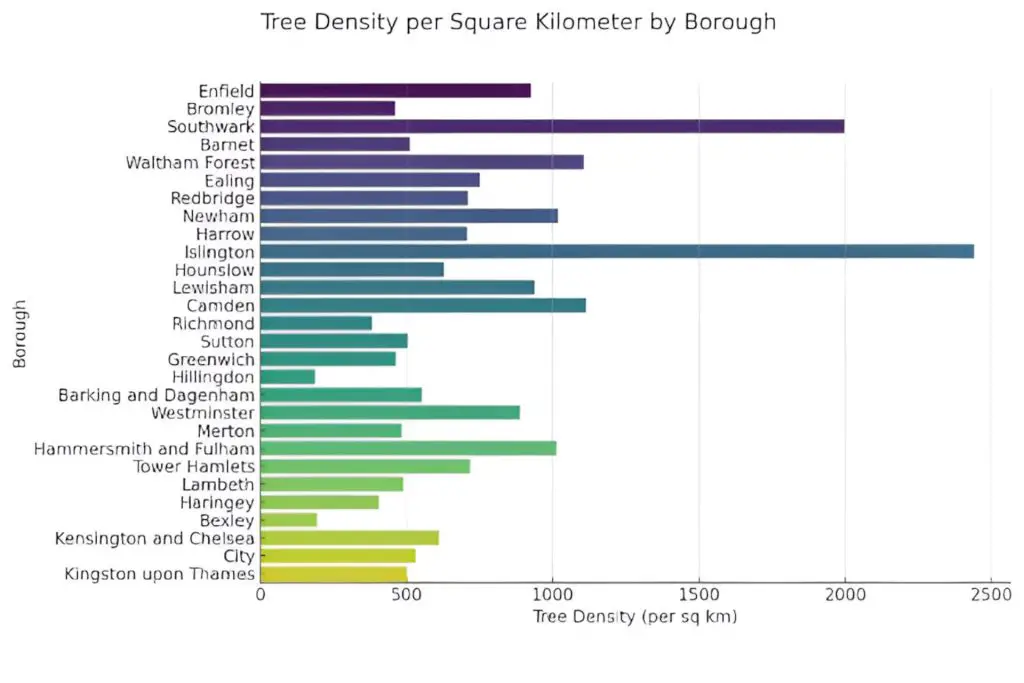
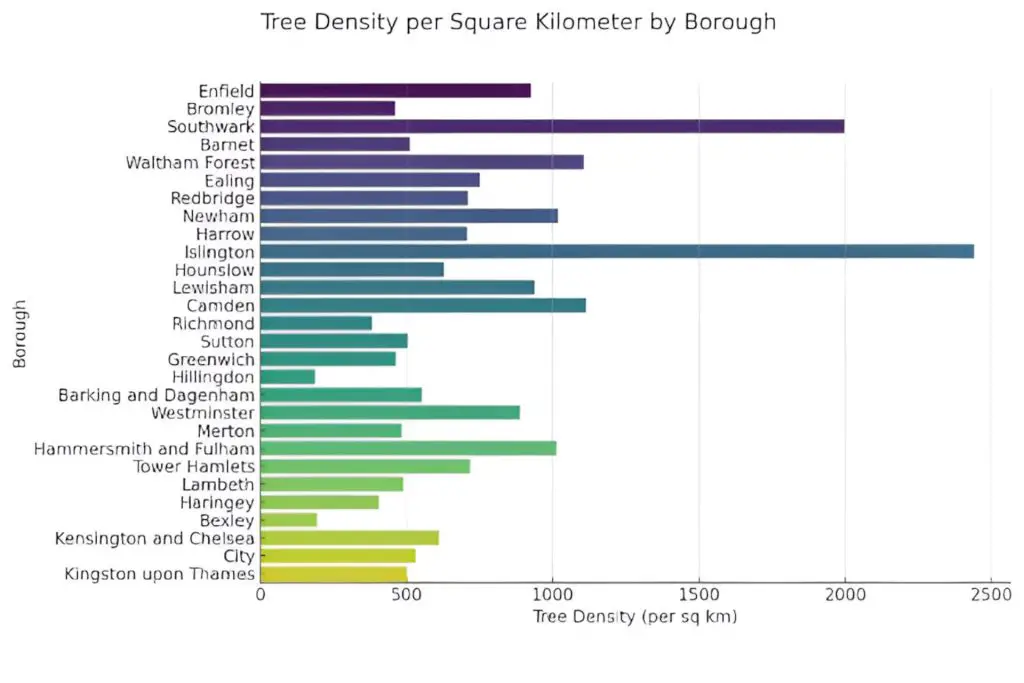
Species Variation
The capacity of trees to absorb carbon dioxide, a climate-regulating service, is not uniform across species. This variation is significant; it shapes the carbon footprint of urban landscapes and informs biodiversity strategies.
Species variation in carbon uptake is a result of several factors, including physiological traits, growth rates, and longevity. Fast-growing species often sequester carbon quickly in their early years but may have a shorter lifespan. Conversely, species with slower growth rates often live longer and accumulate carbon steadily over a more extended period.
For instance, the London Plane tree, a common sight in many cities, is renowned for its tolerance to air pollution and its ability to sequester significant amounts of carbon. On the other hand, species like the Oak and Douglas Fir, although they may grow more slowly, ultimately store vast quantities of carbon due to their large size and longevity.
Deciduous species, often called hardwoods, particularly those that are long-lived are especially effective carbon sinks, while some softwoods, such as red pine and white pine, are also good at storing carbon. Here are some species with high sequestration rates, their characteristics, and how they contribute to carbon management in an urban setting:
- Oak (Quercus spp.)
- Growth: Slow to moderate.
- Longevity: Can live for hundreds of years.
- Sequestration: High due to large biomass and longevity.
- Urban Benefit: Provides extensive canopy for shade and habitat.
- Douglas Fir (Pseudotsuga menziesii)
- Growth: Fast-growing in its youth, slowing with age.
- Longevity: Can live for several centuries.
- Sequestration: Very high due to rapid early growth and substantial final size.
- Urban Benefit: Evergreen, providing year-round carbon uptake and green space.
- Redwood (Sequoia sempervirens)
- Growth: Moderate to fast.
- Longevity: Among the longest-lived tree species.
- Sequestration: Extremely high due to immense size and long life.
- Urban Benefit: Serves as a landmark and supports extensive ecosystems even in urban settings.
- Eucalyptus (Eucalyptus spp.)
- Growth: Very fast.
- Longevity: Shorter lifespan than some other high sequestration species.
- Sequestration: High in the short term due to rapid growth rate.
- Urban Benefit: Quick to provide benefits but may require more frequent replacement.
These species exemplify the principle that ecological services vary distinctly between different tree types. Urban forestry management thus hinges not only on the number of trees planted but also on the strategic selection of species that maximize carbon sequestration and contribute to other ecosystem services, like enhancing urban resilience and supporting wildlife habitats.
To illustrate the comparative sequestration rates, consider the following table:
Species | Sequestration Rate (kg CO2/year) |
---|---|
Oak | 22 |
Douglas Fir | 20 |
Redwood | 25 |
Eucalyptus | 25 |
Maple | 18 |
Pine | 20 |
Birch | 12 |
Urban planners and environmental policymakers must consider these variances to optimize the carbon dynamics of city landscapes. Selecting a diversity of species can also prevent the loss of sequestration capacity due to pests or diseases that might affect a single species.
Incorporating a mix of trees with both high and moderate sequestration rates can ensure a robust and resilient urban forest. This approach balances immediate carbon uptake with sustainable, long-term carbon storage, aligning with goals for carbon neutrality and creating sustainable urban ecosystems.
Age and Maturity
The nexus between the age of trees and their capacity for carbon sequestration is a pivotal aspect of urban forestry management. Trees traverse through life stages, from sapling to maturity, and each stage bears distinct implications for growth rates and carbon dynamics.
In their youth, trees are akin to carbon sponges, vigorously absorbing CO2 as they undergo rapid growth and development. Young trees allocate a substantial portion of the carbon they assimilate into growing new tissues, expanding both their root systems and their above-ground structures. This accelerated growth translates to a higher rate of carbon sequestration per unit of biomass when compared to their elder counterparts.
However, as trees mature, their growth rate decelerates. They enter a phase of carbon equilibrium, where the carbon absorbed through photosynthesis is balanced by the carbon respired back into the atmosphere. Mature trees, with their extensive biomass, store a vast amount of carbon accumulated over the years. Their thick trunks and expansive branches are testament to the decades, sometimes centuries, of carbon storage.
The contrast between young and mature trees in terms of carbon sequestration can be summarized as follows:
- Young Trees:
- High growth rates and rapid carbon uptake.
- Less overall carbon storage due to smaller size.
- Greater susceptibility to environmental stressors and lower overall survival rates.
- Mature Trees:
- Slower growth rates but significant overall carbon storage.
- More resilient to environmental changes and capable of supporting diverse ecosystems.
- Serve as stable carbon reservoirs unless disrupted by disease, disturbance, or death.
A comparative analysis reveals that while young trees are efficient at carbon sequestration on a rate basis, mature trees are unmatched in their total carbon storage capacity. The chart below demonstrates this relationship:
Tree Age | Growth Rate | Carbon Uptake Rate | Total Carbon Storage |
---|---|---|---|
Young (0-10 years) | High | High | Low |
Mature (50+ years) | Low | Moderate to Low | High |
This duality underscores the need for a balanced approach to urban forestry. A diverse age structure within the urban canopy ensures ongoing carbon uptake from young trees, while also preserving the substantial carbon stores held within mature trees.
Urban forestry strategies must also account for the inevitable transition as trees age. Ensuring a continuous pipeline of young trees through planting and effective sapling care is essential. This sustainable cycle fortifies the urban forest’s role in carbon sequestration and enhances its resilience to the impacts of a changing climate.
Further, the age distribution of trees within a city has broader implications for urban ecology. Mature trees provide robust habitats and are key to maintaining biodiversity, while younger trees ensure the regeneration of the urban forest.
Size Matters
The axiom “size matters” is profoundly true in the context of a tree’s ability to sequester carbon. The larger the tree — in height, girth, and canopy spread — the greater its biomass, and consequently, its capacity to store carbon. This relationship between tree size and carbon storage is a cornerstone of urban forestry research and ecosystem service evaluation.
Biomass is the total mass of organic material in a tree — the sum of its wood, leaves, branches, and roots. Since carbon storage is directly proportional to tree biomass, larger trees are more adept at locking away carbon. They stand as massive carbon reservoirs, with the oldest giants encapsulating centuries of atmospheric carbon within their rings.
Estimating the carbon sequestration potential based on tree size involves understanding the biomass-carbon relationship. Typically, a tree’s biomass can be estimated using allometric equations, which relate tree dimensions — such as height and diameter at breast height (DBH) — to their overall mass. Once the biomass is known, a conversion factor (approximately 50% of the biomass is carbon) is used to estimate the carbon content.
The methodology typically involves:
- Measuring Tree Dimensions:
- Height: Measured using tools like clinometers or laser range finders.
- Canopy Spread: Assessed through crown diameter measurements.
- DBH: Measured at 1.3 meters above the ground using a diameter tape.
- Applying Allometric Equations:
- These equations vary by species and region but generally follow the format:
Biomass=a × (DBH) Biomass=a×(DBH)b - Where a and b are coefficients derived from empirical data.
- These equations vary by species and region but generally follow the format:
- Calculating Carbon Storage:
- The estimated biomass is multiplied by the carbon percentage to determine the carbon storage.
By applying this methodology to London’s urban trees, one can create a carbon inventory — a comprehensive account of the carbon sequestered by the city’s green infrastructure. For example, a mature London Plane tree with a DBH of 80 cm might store around 2,400 kg of carbon, whereas a younger specimen with a DBH of 30 cm might store closer to 100 kg.
The following list itemizes typical carbon storage figures for trees of various sizes:
- Small (DBH < 10 cm): 15 – 50 kg of carbon
- Medium (DBH 10-30 cm): 50 – 200 kg of carbon
- Large (DBH > 30 cm): 200 – 2,000+ kg of carbon
These figures illuminate the potential each tree has to combat climate change and affirm the necessity for their protection and incorporation into urban landscapes.
Tree Health and Vigor
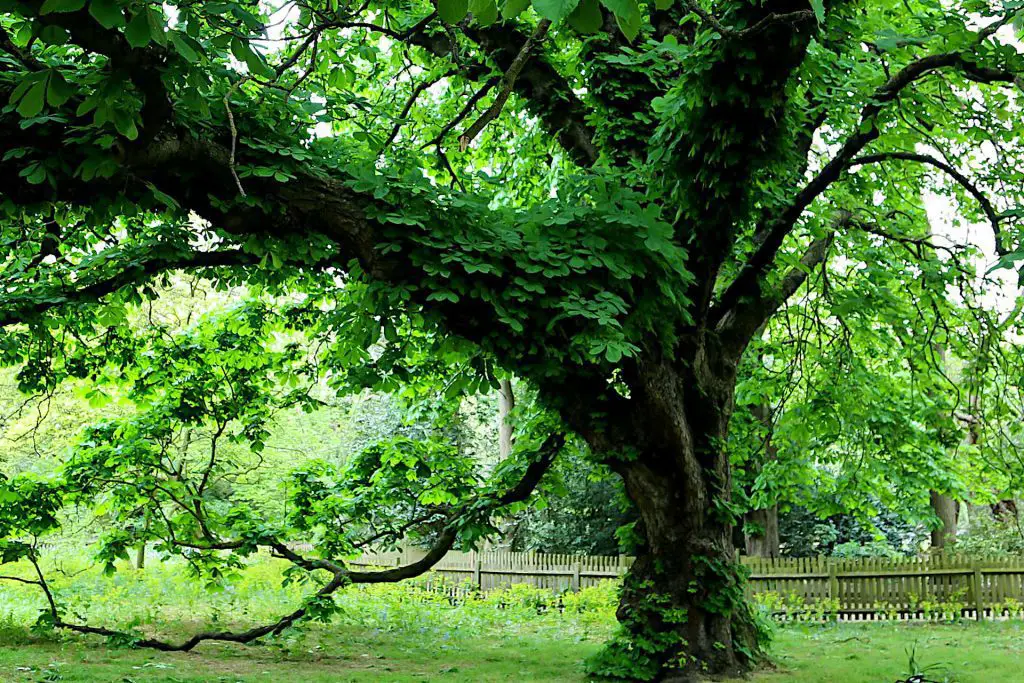
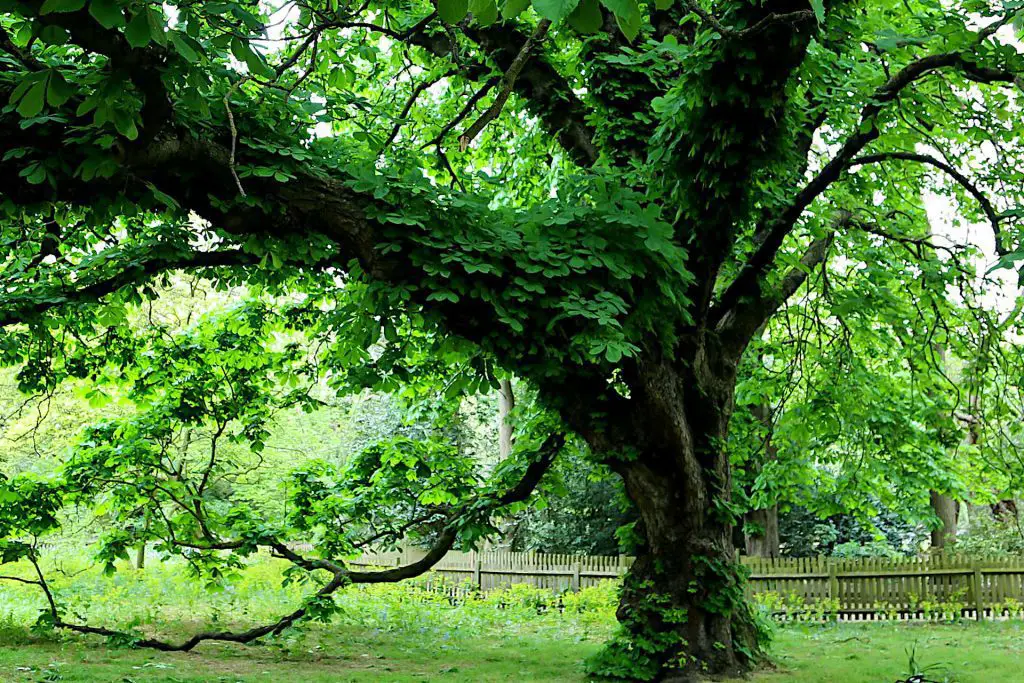
The health and vigor of a tree are paramount to its carbon sequestration efficiency.3 Just as the well-being of a human influences its capacity to perform, the physiological state of a tree determines its ability to partake in carbon assimilation. Stressed or diseased trees exhibit reduced photosynthetic activity, impairing their carbon uptake and storage abilities.
A healthy tree, with its rich canopy and robust root system, not only grows more vigorously but also possesses a heightened capacity for CO2 absorption. The photosynthesis process — the tree’s mechanism for converting sunlight into energy — is optimized when the tree is free from afflictions such as pests, pathogens, or environmental stressors.
Tree health can be gauged through various indicators:
- Foliage: Healthy trees display full, vibrant canopies. Discoloration or premature leaf drop can signal distress.
- Bark Integrity: Damaged or decaying bark can be an entry point for disease.
- Root Health: Visible, surface-level roots should be firm and free from decay.
- Growth Patterns: Uniform growth rings indicate consistent, healthy growth.
Maintaining tree health for maximum carbon absorption involves a series of proactive measures:
- Regular Monitoring: Early detection of health issues is critical for timely intervention.
- Pest and Disease Management: Implementing control measures to manage outbreaks that can compromise tree health.
- Soil Care: Ensuring adequate soil conditions for nutrient uptake and root development.
- Pruning: Removing dead or diseased limbs to promote healthy growth and canopy structure.
- Watering: Providing sufficient water, especially in times of drought, to avoid stress.
Investment in these practices sustains not only the individual tree but the entire urban canopy, bolstering the ecosystem’s overall sequestration potential. For example, a healthy mature oak tree can sequester up to 22 kg of carbon annually, whereas one in poor health may sequester significantly less, if at all.
The following table outlines the potential impact of health factors on carbon sequestration rates:
Health Factor | Potential Impact on Carbon Sequestration |
---|---|
Full Canopy | +15-25% |
Pest-Free | +10-20% |
Adequate Water Supply | +20-30% |
Healthy Soil Conditions | +20-25% |
The sustainability of an urban forest hinges on the health of its constituent trees. Vigorous, thriving trees are not only more capable of sequestering carbon but also provide a multitude of additional benefits such as habitat provision, urban heat island mitigation, and recreational spaces for communities. They enhance the aesthetic value of the urban landscape, contribute to biodiversity, and improve the quality of life for city inhabitants.
In essence, the vigor of each tree within the urban fabric is a critical component of the broader climate change mitigation strategy. It is a testament to the value of preserving and nurturing these natural assets, ensuring that they continue to serve as vital components of the urban ecosystem, combating carbon emissions one leaf at a time.
Density and Forest Cover
Tree density and the extent of urban forest cover are critical metrics in the quest to enhance carbon sequestration. A higher density of trees per unit area usually translates to greater overall carbon uptake, making the optimization of urban spaces for tree planting not just a landscaping priority but a climate action imperative.
Tree density influences the urban canopy’s ability to act as a carbon sink in several ways. Densely planted areas can sequester more CO2 simply due to the number of trees present. Moreover, a well-planned array of diverse species can maximize space use and carbon absorption while minimizing competition for sunlight and resources.
Urban forest cover, or the proportion of an area shaded by tree canopies, is indicative of the urban forest’s health and its potential to impact the urban microclimate. Increased canopy cover can reduce the heat island effect, lower energy consumption, and contribute to the overall carbon sequestration of the region.
To optimize urban spaces for tree planting and maximize their sequestration potential, the following strategies can be employed:
- Green Infrastructure Integration: Incorporating tree planting into the design of new developments and infrastructure projects.
- Vertical Greening: Utilizing vertical spaces on buildings for planting, which can also contribute to carbon sequestration.
- Rooftop Gardens: Encouraging the installation of green roofs that not only sequester carbon but also provide additional insulation.
- Community Greening Initiatives: Engaging communities to participate in tree planting and care, fostering stewardship and expanding green cover.
- Street Tree Expansion: Increasing the number of street trees, requires careful species selection to ensure resilience and minimize maintenance.
A key aspect of implementing these strategies is the need for urban planning to prioritize green spaces amid the competing demands for development. Trees need to be regarded as essential urban infrastructure, as vital as roads or utilities.
Consider the following illustrative figures demonstrating the importance of tree density and forest cover:
- An increase of 10% in urban tree cover has the potential to enhance overall carbon sequestration by up to 15%.
- A city with 30% canopy cover might possibly sequester 50% more carbon annually than a city with only 20% cover.
The synergistic effect of increased tree density and urban forest cover cannot be overstated. A dense and healthy urban forest is a multi-functional asset that provides ecological, economic, and social benefits, contributing significantly to urban sustainability and resiliency.
A Comparative Analysis:
In the diverse tapestry of London’s urban ecology, certain boroughs have emerged as sequestration champions, setting benchmarks for carbon absorption through strategic tree management and urban forestry initiatives. These boroughs, with their high sequestration rates, exemplify the successful integration of green infrastructure into urban planning.
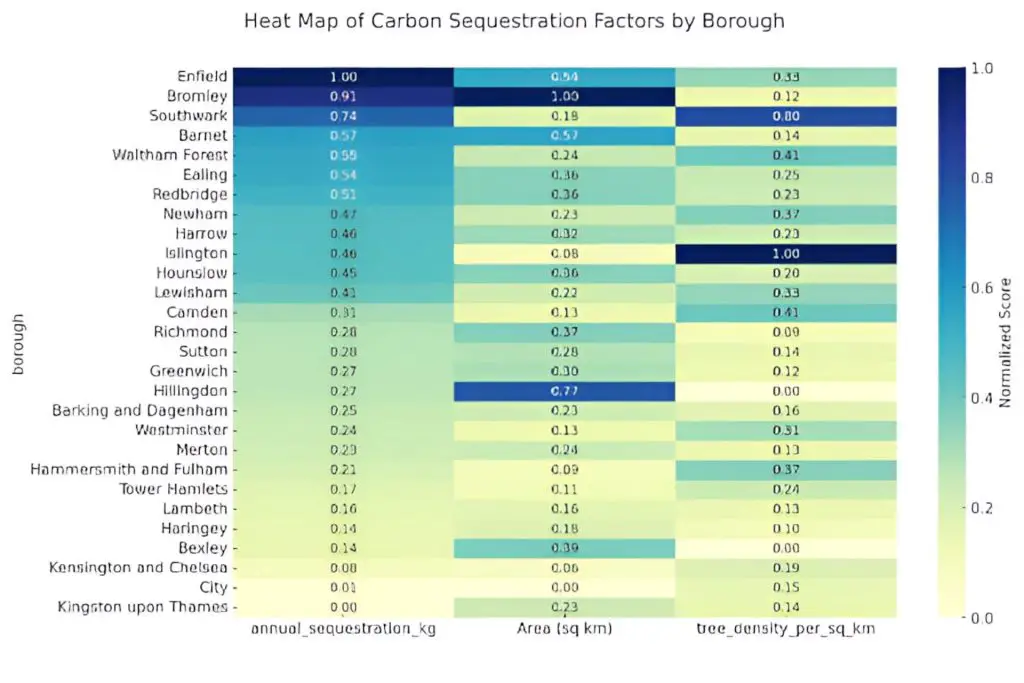
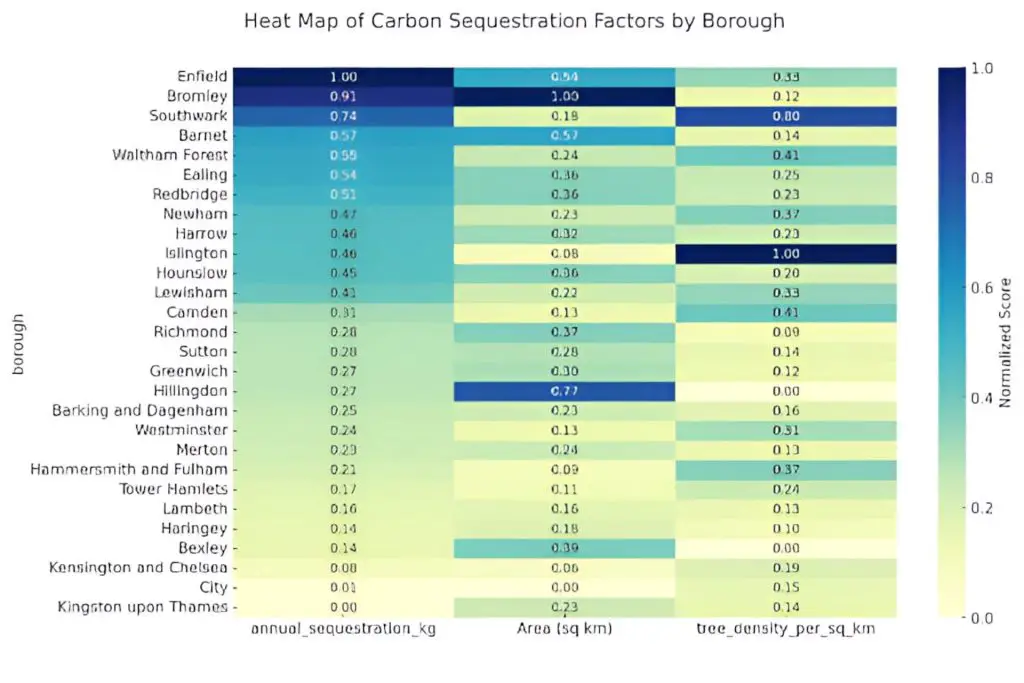
Leading the Way: Borough of Enfield
With an estimated 80 million kilograms of carbon dioxide sequestrated annually, the borough of Enfield’s, strategic approach to urban forestry has yielded impressive results, positioning it as a model of ecological stewardship within London’s metropolitan landscape. Enfield’s success in this domain can be attributed to a confluence of factors, including its commitment to species diversity, tree density, and community engagement.
Enfield’s tree inventory showcases a rich tapestry of species, each playing a role in the carbon cycle. From the towering London Plane to the robust Oak, these trees represent a carefully curated selection aimed at maximizing carbon uptake. Such diversity not only enhances the aesthetic value of the borough’s green spaces but also fortifies the urban forest against disease and climate-related stresses.
The borough’s tree density strategy reflects an understanding of the spatial dynamics of urban planning. Enfield has managed to maintain high tree densities in both residential and industrial areas, intertwining green infrastructure with urban development. The density of the urban canopy in Enfield is a testament to the symbiotic relationship between urban living and natural ecosystems.
The following table illustrates Enfield’s achievements in species diversity and tree density:
Indicator | Enfield’s Performance |
---|---|
Species Diversity | High – over 50 distinct species |
Tree Density | High – 2,500 trees per square kilometer |
Diversity in Density: Stand-Out Boroughs
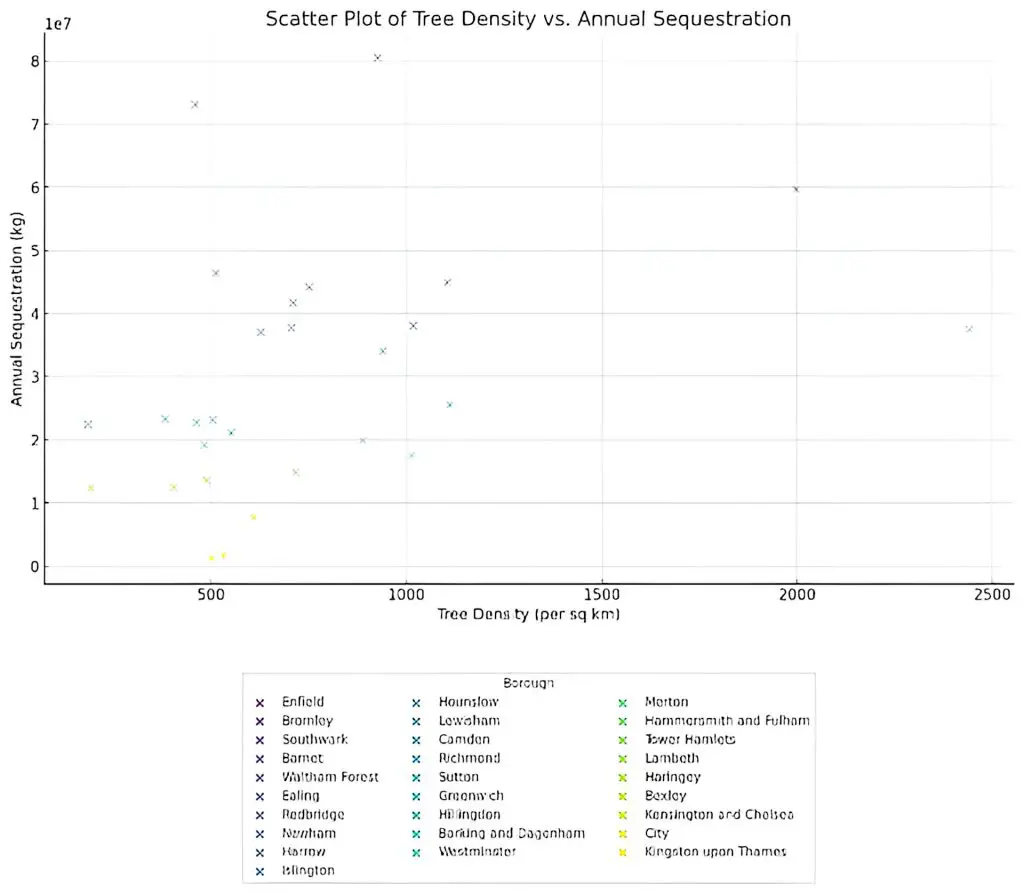
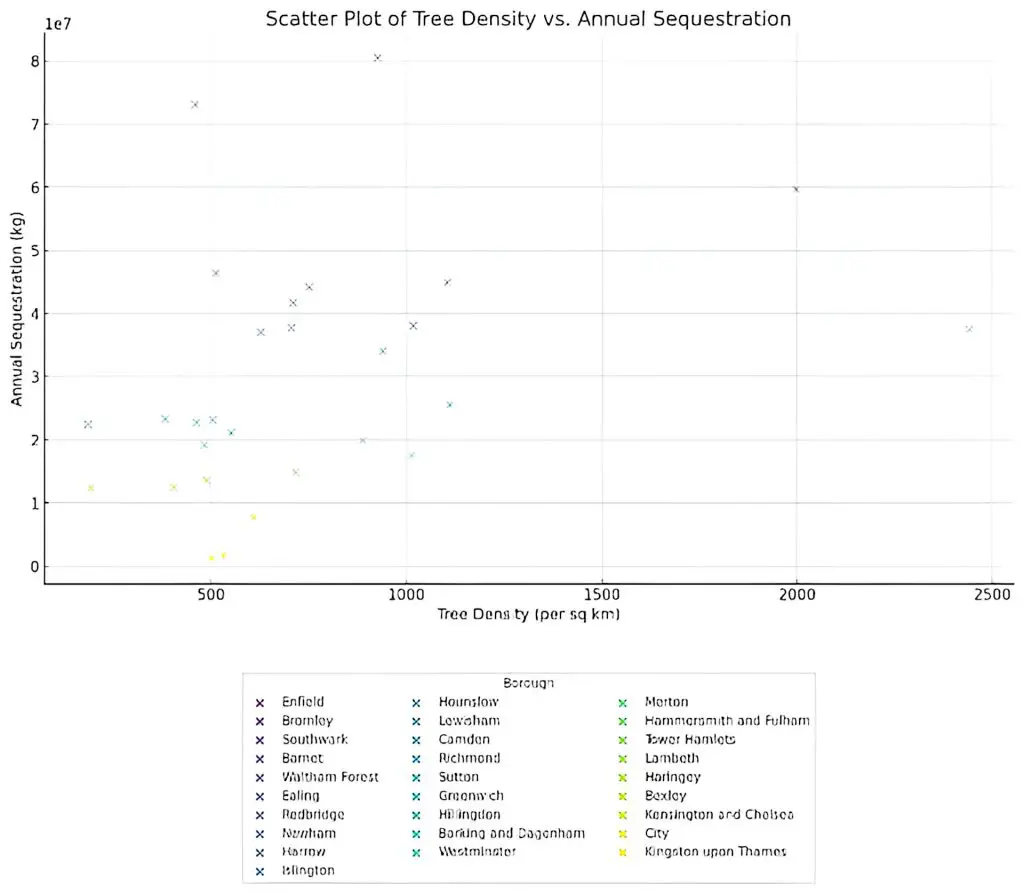
Besides Enfield, there are several other stand-out boroughs. An examination of tree density figures across London’s boroughs reveals a mosaic of green infrastructure, each with varying implications for carbon sequestration and urban environmental quality. Tree density, defined as the number of trees per unit area, is a critical factor influencing not only the aesthetic appeal of urban spaces but also their ecological functionality.
Boroughs like Islington and Hammersmith and Fulham, with higher built density, face challenges in achieving high tree densities due to limited available planting space. Conversely, boroughs with more open spaces, such as Richmond upon Thames and Barnet, although having lower tree densities, still have good carbon sequestration capabilities.
The relationship between tree density and urban planning is complex and multifaceted. Urban planning decisions directly affect tree density by dictating land use and the allocation of space for green areas. To foster higher tree densities, urban planners must:
- Integrate Trees in Development Plans: Ensuring that new developments include plans for tree planting and green space.
- Adopt Tree Preservation Regulations: Implementing policies that protect existing trees and require replacements for those removed.
- Promote Innovative Greening Solutions: Encouraging the use of green roofs, walls, and other structures to supplement ground-level tree planting.
- Leverage Community Spaces: Utilizing schools, parks, and communal areas for tree planting, engaging communities in the process.
- Incentivize Private Planting: Offering incentives for private landowners to plant and maintain trees on their properties.
Tree density not only impacts carbon sequestration but also affects biodiversity, air quality, and the well-being of urban residents. The following table provides a comparative analysis of tree density figures and their implications for select boroughs:
Borough | Tree Density (Trees per km²) | Carbon Sequestration (kg CO₂/year) | Urban Planning Implications |
---|---|---|---|
Islington | 2441 | 37,489,970 | Need for vertical greening and rooftop gardens due to limited space. |
Hammersmith and Fulham | 1,013 | 17,546,120 | Need to incentivize private planting and promote more innovative solutions. |
Richmond upon Thames | 384 | 23,294,070 | Utilization of parklands and open spaces for sequestration enhancement. |
Barnet | 513 | 46,405,000 | Balancing suburban expansion with the preservation of green corridors. |
This diversity in tree density underscores the need for tailored urban forestry strategies that reflect the unique characteristics of each borough. Effective urban planning must harmonize the need for development with environmental sustainability, recognizing trees as vital components of the urban ecosystem.
Boroughs with lower tree densities must innovate to overcome spatial constraints, while those with higher densities must ensure that tree health and diversity are maintained. In every case, the goal is the same: to optimize the urban landscape for both human habitation and ecological services, such as carbon sequestration, that trees provide.
Species Strategy
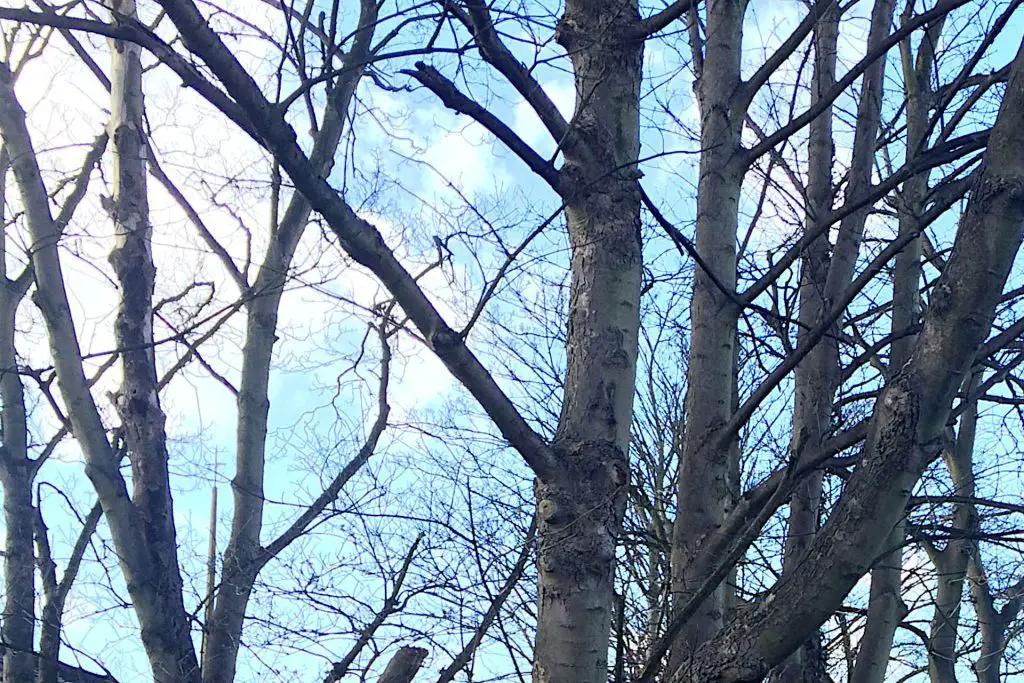
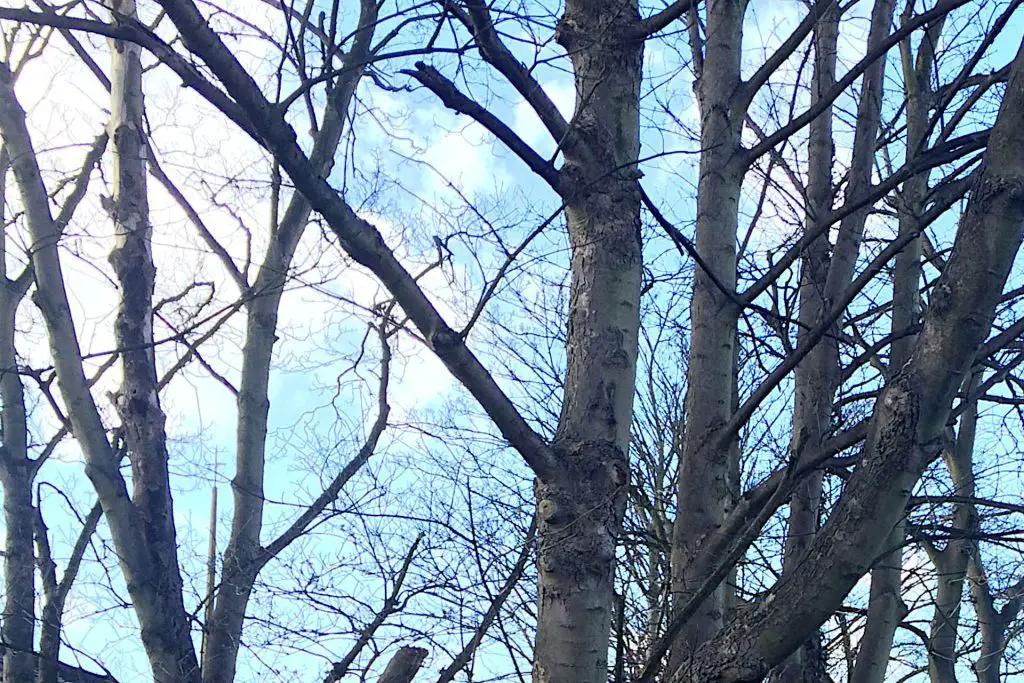
the selection of tree species is a deliberate strategy that significantly influences carbon sequestration. Each species has a unique set of characteristics, such as growth rate, biomass accumulation, and longevity, which determine its carbon storage capacity. London’s boroughs, with their varied approaches to species selection, provide a living laboratory for understanding the impact of these choices on urban carbon dynamics.
Species strategy is not a one-size-fits-all endeavor; it requires a nuanced understanding of local climate, soil conditions, and the urban fabric. For instance, the London Plane tree, celebrated for its resilience to pollution, is a common sight in many boroughs and a prime contributor to carbon sequestration. However, boroughs like Camden and Islington have also begun to diversify their species portfolio to increase resilience and carbon sequestration rates.
Effective species-specific planting strategies are exemplified in boroughs such as:
- Greenwich: Known for its historical landscapes, the borough has embraced a mix of native species like Oaks and Horse Chestnuts, which are not only culturally significant but also adept at carbon storage.
- Southwark: Here, the emphasis on species diversity has led to an urban forest that is not only more resilient to pests and diseases but also optimizes carbon sequestration across different tree species with varying rates of carbon uptake.
The impact of species selection on carbon sequestration is highlighted in the following case studies:
- Richmond upon Thames’ Oak-Led Strategy:
- Oaks are known for their substantial biomass and carbon sequestration capabilities.
- The borough’s focus on Oak planting has led to an estimated increase of 15% in carbon sequestration over the past decade.
- Lewisham’s Diverse Urban Canopy:
- By planting a variety of species, Lewisham has created a robust urban forest that mitigates the risk of species-specific diseases and maximizes carbon absorption.
- The strategy has contributed to an urban forest that sequesters an estimated 20% more carbon than if a single-species strategy had been employed.
Note: The percentage increases are indicative based on urban forestry studies.
These strategies are not just about planting trees; they are about planting the right trees in the right places for maximum ecological benefit. The table below offers a snapshot of the carbon sequestration impact of different species strategies:
Borough | Dominant Species | Estimated CO2 Sequestration Impact |
---|---|---|
Greenwich | Oaks, Horse Chestnuts | High biomass contribution to carbon storage |
Southwark | Diverse species | Risk mitigation and optimized carbon uptake |
Richmond upon Thames | Oaks | Enhanced sequestration through large biomass trees |
Lewisham | Species diversity | Increased overall sequestration due to diversity |
In essence, species strategy is a key facet of urban forestry management that directly impacts the carbon sequestration profile of a borough. By employing strategic species selection, boroughs can enhance their contribution to climate mitigation efforts. A thoughtful species strategy, informed by environmental science and urban ecology, ensures that London’s urban forests continue to thrive and contribute to a greener, more sustainable cityscape.
London’s Boroughs: Challenges and Opportunities
Data Gaps
In any empirical study, particularly those involving environmental metrics such as carbon sequestration, data integrity is paramount. The analysis of London’s boroughs is not exempt from these challenges. Acknowledging the data gaps and the consequent exclusion of certain boroughs from the comprehensive analysis is critical in painting an accurate picture of the urban forestry landscape and its potential to mitigate carbon emissions.
Boroughs such as Brent, Croydon, Havering, Hackney, and Wandsworth were notably excluded due to substantial gaps in their tree inventory data. These gaps include incomplete records of tree species, ages, and conditions — key variables that significantly influence carbon sequestration rates. The absence of this information hinders our ability to make informed decisions and to fully understand the ecosystem services provided by the urban forest.
The need for comprehensive, high-quality data is a cornerstone of effective urban forestry strategies. Detailed and accurate data inform several critical areas:
- Species-Specific Sequestration Rates: Understanding which tree species are most effective at carbon uptake in specific urban environments.
- Tree Health and Vigor: Monitoring the health of the urban canopy to ensure trees are sequestering carbon efficiently and are not at risk of disease or decline.
- Demographic Distribution: Mapping the age and maturity of trees to anticipate growth patterns and sequestration potential over time.
- Impact Assessment: Measuring the real-time impact of urban forestry initiatives on carbon sequestration to justify funding and policy support.
The following list highlights the key types of data required for a holistic analysis:
- Tree Density: The number of trees per unit area in each borough.
- Biomass Estimates: The amount of carbon stored in the trees of each borough.
- Growth Rates: How quickly trees in each borough are sequestering carbon.
To address these gaps, a multidisciplinary approach combining remote sensing technology, field surveys, and community science initiatives could be utilized. This approach would ensure that the data collected is not only comprehensive but also continuously updated and verified.
The potential benefits of closing these data gaps are manifold. With complete data sets, urban planners and environmental scientists could create adaptive management plans that are responsive to the changing needs of the urban ecosystem. Additionally, it would allow for the development of predictive models to forecast how changes in urban forestry strategies could impact carbon sequestration in the future.
The table below illustrates a hypothetical scenario where data gaps are addressed:
Data Type | Current Status | Potential Status with Data Improvement |
---|---|---|
Tree Density | Incomplete data for some boroughs | Complete census enabling accurate density calculations |
Biomass Estimates | Estimated values with high uncertainty | Precise measurements improving carbon budgeting |
Growth Rates | Generalized rates applied | Species and age-specific rates tailored to local conditions |
While data gaps clearly present significant challenges to our understanding and management of urban forests, they also offer opportunities. By prioritizing data collection and quality, London can lead by example in the global effort to enhance urban greenery and its role in climate change mitigation. Bridging these gaps is not merely an academic exercise; it is a practical necessity for the future health of our cities and the planet.
Urban Forestry Management
Urban forestry management in densely populated cities like London contends with a unique set of challenges. The task of maintaining, protecting, and expanding urban forests is a complex interplay of environmental science, public policy, and community engagement.
Land Availability is a primary concern. In cities where every square foot of land is valuable, dedicating space for trees competes with housing, infrastructure, and commercial development. The scarcity of open land necessitates creative solutions for integrating trees into the urban matrix.
Climate Adaptability is another hurdle. As climate patterns shift, urban forestry must adapt to ensure tree species can thrive in new conditions. This includes accounting for increased risks from pests, diseases, and weather extremes.
The Economic Constraints faced by municipal budgets cannot be understated. Urban forestry programs often must justify their costs against other critical urban services, requiring clear demonstration of their long-term economic and environmental benefits.
Despite these challenges, potential solutions and innovative approaches abound:
- Vertical Greening: Implementing green walls and roof gardens to enhance green space without expanding the urban footprint.
- Green Corridors: Connecting parks and green spaces to create a continuous network that facilitates wildlife movement and enriches biodiversity.
- Community Forestry: Engaging local residents in the planting and care of urban trees, fostering a sense of ownership and stewardship.
- Public-Private Partnerships (PPPs): Leveraging private sector resources and expertise to fund and manage urban forestry projects.
- Climate-Smart Selection: Choosing tree species that are resilient to changing climate conditions and can provide the greatest environmental benefits.
One innovative example is the Urban Tree Challenge Fund, a program designed to incentivize tree planting in urban areas with limited green space. This fund has catalyzed the planting of thousands of trees in London, contributing to the city’s carbon sequestration goals.
A table illustrating the impact of innovative urban forestry solutions in London might look like this:
Innovation | Description | Impact on Urban Forestry Management |
---|---|---|
Vertical Greening | Utilization of vertical surfaces for planting | Expands green space without increasing land use |
Green Corridors | Networks of green spaces for ecological connectivity | Enhances biodiversity and ecosystem services |
Community Forestry | Engagement of local communities in tree planting and care | Increases tree survival rates and fosters environmental stewardship |
PPPs | Collaboration with the private sector | Brings additional funding and innovation to urban forestry projects |
Climate-Smart Selection | Selection of resilient tree species | Ensures long-term sustainability of urban forests |
Contribution to London’s Carbon Neutrality Goals
Urban forests are pivotal in the city’s quest for carbon neutrality. Through photosynthesis, trees absorb carbon dioxide, offsetting emissions from other sources. The potential of urban forests to contribute to London’s carbon neutrality is substantial, with the following benefits:
- Reduction of the Urban Heat Island Effect: Trees cool the air, reducing energy consumption for air conditioning.
- Improvement of Air Quality: Trees act as natural air filters, removing pollutants from the environment.
- Enhancement of Biodiversity: Urban forests provide habitats for wildlife, supporting ecological balance.
- Carbon Storage: Mature trees can store significant amounts of carbon over their lifetimes.
- Noise reduction: Environmental noise can be greatly reduced as trees can play a significant role in mitigating urban noise
To visualize the impact of informed policies on urban forestry, consider the following hypothetical projection:
Year | Policy Implementation | Projected Increase in Carbon Sequestration |
---|---|---|
2025 | High | 5% increase due to new planting initiatives |
2030 | Moderate | 10% increase with the maturity of new plantings |
2035 | Low | Stagnation due to lack of policy reinforcement |
Final Thoughts
The exploration of London’s urban forests has shed light on the vital role that trees play in mitigating carbon emissions. Amidst the concrete and steel of city landscapes, trees stand as natural allies in the battle against climate change. Through the process of carbon sequestration, they absorb carbon dioxide, one of the primary greenhouse gases, and store carbon in their wood, leaves, and soil, making urban forestry a key component in the pursuit of environmental sustainability.
However, the journey does not end with the planting of trees. Ongoing research is essential to deepen our understanding of urban forestry dynamics and to enhance the efficacy of trees as carbon sinks. Data-driven insights are crucial for tailoring tree-planting programs that optimize carbon sequestration, and for developing maintenance practices that ensure the longevity and health of urban trees.
Tables
Boroughs by Estimated Rank for Carbon Sequestration
Rank | Borough | Annual Sequestration (kg) | Area (sq km) | Population | Tree Density (per sq km) |
---|---|---|---|---|---|
1 | Enfield | 80,471,730 | 82.21 | 333,794 | 926.68 |
2 | Bromley | 73,058,130 | 150.14 | 332,336 | 459.11 |
3 | Southwark | 59,626,000 | 28.85 | 318,830 | 1997.71 |
4 | Barnet | 46,405,000 | 86.74 | 395,896 | 512.54 |
5 | Waltham Forest | 44,889,280 | 38.82 | 276,983 | 1104.83 |
6 | Ealing | 44,169,000 | 55.53 | 341,806 | 751.85 |
7 | Redbridge | 41,675,060 | 56.41 | 305,222 | 710.74 |
8 | Newham | 38,042,310 | 36.21 | 353,134 | 1017.48 |
9 | Harrow | 37,697,780 | 50.48 | 251,160 | 706.30 |
10 | Islington | 37,489,970 | 14.87 | 242,467 | 2440.98 |
11 | Hounslow | 37,016,020 | 55.97 | 271,523 | 628.04 |
12 | Lewisham | 34,026,580 | 35.15 | 305,842 | 940.42 |
13 | Camden | 25,525,340 | 21.76 | 270,029 | 1111.47 |
14 | Richmond | 23,294,070 | 57.42 | 198,019 | 383.98 |
15 | Sutton | 23,146,410 | 43.85 | 206,349 | 504.49 |
16 | Greenwich | 22,713,390 | 47.35 | 287,942 | 463.43 |
17 | Hillingdon | 22,426,330 | 115.69 | 306,870 | 185.60 |
18 | Barking and Dagenham | 21,085,570 | 36.08 | 212,906 | 552.21 |
19 | Westminster | 19,880,590 | 21.47 | 261,317 | 888.50 |
20 | Merton | 19,166,450 | 37.61 | 206,548 | 483.50 |
21 | Hammersmith and Fulham | 17,546,120 | 16.39 | 185,143 | 1013.38 |
22 | Tower Hamlets | 14,838,710 | 19.76 | 324,745 | 717.35 |
23 | Lambeth | 13,571,810 | 26.83 | 326,034 | 489.11 |
24 | Haringey | 12,466,000 | 29.58 | 268,647 | 404.87 |
25 | Bexley | 12,383,790 | 60.55 | 248,287 | 193.22 |
26 | Kensington and Chelsea | 7,692,733 | 12.12 | 156,129 | 610.09 |
27 | City | 1,616,584 | 2.90 | 9,721 | 532.27 |
28 | Kingston upon Thames | 1,161,419 | 37.24 | 177,507 | 502.15 |
Carbon Sequestration Rate By Tree Type
Tree Species | Carbon Sequestration Rate (t CO2/ha/year) |
---|---|
Alder | 10 |
Aspen | 12 |
Beech | 15 |
Birch | 10 |
Cedar | 15 |
Douglas fir | 20 |
Elm | 10 |
Eucalyptus | 25 |
Fir | 15 |
Hemlock | 10 |
Hickory | 12 |
Maple | 15 |
Oak | 15 |
Pine | 20 |
Redwoods | 25 |
Spruce | 15 |
Sycamore | 10 |
Willow | 15 |
References
- Natural England Research Report NERR094: “Carbon Storage and Sequestration by Habitat 2021“
- Data.gov.uk: “Local Authority Maintained Trees“
- PLOS Journal:”The impact of tree age on biomass growth and carbon accumulation capacity“
- US Forest Service: “CUFR Tree Carbon Calculator (CTCC)“